In her forays into physics, University of Cambridge physicist Suchitra Sebastian heads for the hinterlands, hoping to be the first to leave footprints.
"I like to explore unknown territory," she said.
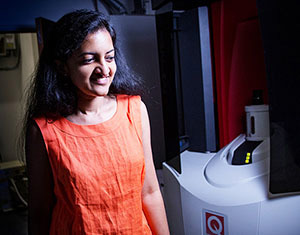
Suchitra Sebastian
Photo by: Phillipp Ammon/Quanta Magazine
Which is not to say she's wandering blindly into the science haystack. She has a definite strategy about where the coolest physics needles are hiding.
Sebastian studies correlated electron systems — materials in which electrons can team up and behave radically differently from individual electrons. Recently, Sebastian has set her sites on samarium hexaboride (SmB6). Some physicists who have studied that system have proposed that it belongs to a class of materials called topological insulators.
Discovered in 2007, topological insulators are the split personalities of materials: They conduct electricity in some areas, but don't in others. In topological insulators that are two-dimensional (only one atomic layer thick), current runs only along the edges, like a racecar circling a track while avoiding the infield, which acts as an insulator. In 3-D topological insulators, current can run anywhere on the surface, but not through the interior (called the "bulk" by physicists). Picture that racecar speeding along any road on Earth, but never penetrating the planet.
(If the term "topological" rings a bell, it might be because the 2016 Nobel Prize in Physics went to a trio of scientists who applied concepts of topology, a branch of mathematics, to better explain and predict so-called "exotic" states and transitions of matter.)
Sebastian set out to try and establish the topological character of SmB6 by looking at how electrons travel through its conducting surface. As electrons propagate through a conductor, their orbits forge a rather zigzagging path. Because SmB6 was a supposed topological insulator, Sebastian expected that those orbits would be restricted to the very surface of the material — that their path would be strictly two-dimensional.
But what Sebastian and her group found during experiments in 2015 was far stranger. When they studied SmB6 using a very high magnetic field, they found what appeared to be large, three-dimensional electron orbits. That would mean that the electron orbits were moving through the interior of the material, which is an insulator.
"My first reaction was, 'Wow, something strange is happening,'" said Sebastian, who was conducting the experiment at the National MagLab at the time. "How could we be seeing large electron orbits in the bulk? The electrons in the bulk of SmB6 shouldn't be moving at all — the bulk is insulating!"
She phoned a colleague at Cambridge for his thoughts. "You do realize," he insisted, "this is impossible."
Apparently not.
Further afield
Sebastian has since been trying to figure out this unusual effect — a material that appears to behave simultaneously as an insulator and a conductor. Is it a never before observed quantum phase of matter? Could it be that electrons behaving collectively (known as quasiparticles) in this strange quantum phase act completely unlike individual electrons?
"Perhaps the quasiparticles we see orbiting are starkly different from electrons or electron-like objects," Sebastian suggested. "We begin to consider the radical possibility that perhaps SmB6 defies conventional understanding, and comprise neutral quasiparticles — ones that, unlike electrons, carry no charge!"
In her search for answers, Sebastian sticks to the fringe. She focuses on critical phase transition regions — the thresholds between states of matter that occur at specific pressures or temperatures, like water boiling as it turns into steam under a critical pressure.
"We are interested in the quantum version of this critical regime, where a growth of quantum fluctuations means that the interactions between electrons grow extremely large, mediating new and exotic quantum phases," she said. "This region is therefore an incredibly exciting region that is ripe for exploration."
By Kristen Coyne
This story was originally part of a series of stories about Science on the Edge. To read the other stories in the series, see Bursting the Oil Water Bubble, Safer Lithium Batteries and Border Patrol.