"No man is an island," English poet John Donne wrote almost 500 years ago. Neither, to put a modern, scientific twist on that famous line, are the things man is made of: cells.
Cells are surrounded by a protective wall called the lipid bilayer. But they still, at that interface, need to interact with the outside world.
That's the job of membrane proteins, the bouncers of the cellular world. They police what comes in and what leaves while keeping an eye out for trouble.
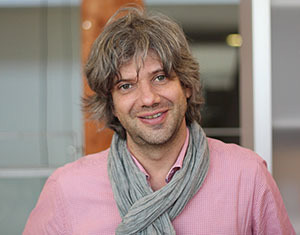
Guido Pintacuda
Photo by: Loren Andreas and Michael J. Knight
"They're key players in allowing cells to adapt to new living conditions or to protect the cell from certain events happening outside," explained Guido Pintacuda, research director at the Center for High Field Nuclear Magnetic Resonance (NMR) in Lyon, France. "For example, they are able to communicate to the outside world a change which is happening inside the cell. This immediately makes them important drug targets."
In fact, about 40 percent of all FDA-approved drugs are designed to manipulate membrane proteins in some way.
Yet they remain largely a mystery. Despite their numbers (they make up about 20 to 30 percent of all living proteins) and the pivotal roles they play in our bodies, less than 2 percent of all the proteins scientists have characterized to date are membrane proteins.
That's because those boundary-dwellers are often in charge of complex tasks and need a complex structure to execute them. Typically they contain several hundred amino acids arranged in rigid scaffolds alternating with less-ordered portions.
Scientists also face big technical hurdles with the NMR instruments they use to understand what these proteins look like and how they function. But groups such as Pintacuda's are developing new tricks to make solid-state NMR a more powerful and versatile tool for the job.
"Increasingly it's the technique of choice," he said.
Using solid-state NMR, scientists can map out the structure of complex macromolecules by using magnetic fields and radio waves that exploit magnetic properties inherent to all atoms. Depending on the specific technique applied, the result is either a "snapshot" detailing the molecule's structure or a kind of movie that reveals how it behaves in its native environment.
"We can see what happens to each atom of a large molecule over time," Pintacuda said. "We can look at the molecule in three dimensions as it if was at the cinema, and we see how it functions."
One protein much scrutinized by scientists working at the Francis Bitter Magnet Lab at MIT, the National MagLab and other labs is known as the M2 proton channel. Located in the wall of the influenza A virus, it ushers protons in and out of the cell. Tim Cross at the National MagLab discovered how the protein functions, and his and other groups are identifying new drugs to block it, thus preventing or thwarting infection.
At the Center for High Field NMR in Lyon, Pintacuda’s group has helped its collaborators in studying a mutated form of that protein on a drug-resistant version of influenza A, shedding light on how the mutation allows the M2 proton channel to keep working even after exposure to drugs.
Their NMR research would not be possible without high magnetic fields. The stronger their magnets, the better NMR systems can "hear" and separate the tiny signals emitted by different atoms in the molecules they're listening to. One might imagine NMR like an elephant, an animal renowned for excellent hearing, thanks to its big, amplifier-like ears. Dr. Seuss fans might even bring to mind the author’s famous pachyderm, Horton, whose sensitive ears could make out voices of the residents of Whoville, who inhabited a speck of dust.
"We're able to isolate the voice of each individual atom in the molecule," said Pintacuda, "and therefore much better characterize what’s happening at atomic resolution."
By Kristen Coyne
This story was originally part of a series of stories about Science on the Edge. To read the other stories in the series, see Bursting the Oil Water Bubble, Safer Lithium Batteries and Surprise Under the Surface.