Laboratories are things; they don't have feelings. But if they did, the half dozen high-field magnet labs around the world could be forgiven for feeling a little envious of CERN (the European Organization for Nuclear Research), whose budget and name recognition dwarf their own. To add insult to injury, some less informed visitors to magnet labs often ask about the particle physics research occurring there. That's kind of like raving to Stephen Baldwin, Alec's lesser-known thespian brother, about how much you love "30 Rock." (For the record, physicists at magnet labs generally study condensed matter physics — the quantum mysteries of solid materials — rather than hadrons and quarks.)
CERN's science celebrity is understandable. After all, that's where the long-predicted Higgs boson was observed, a Nobel Prize-winning physics landmark. Its Large Hadron Collider (LHC), with a 27-kilometer (about 17 miles) circumference, is the biggest instrument ever built for scientific research, straddling two countries. Half of the world's particle physicists work at CERN. They even founded the World Wide Web!
Yet behind any moonshot-scale enterprise like CERN stands a vast cast of players, and magnet labs play a key role. The European lab is now in the midst of a $1-billion, decade-long upgrade to the LHC, known as the High-Luminosity LHC (HL-LHC or HiLumi for short), an effort that involves reinventing some of the thousands of superconducting magnets critical to its operation. For this effort CERN, in partnership with the U.S. Department of Energy (DOE), has been tapping the expertise of magnet R&D specialists at the National High Magnetic Field Laboratory in Florida.
Beyond HiLumi looms CERN's even more ambitious proposed Future Circular Collider (FCC), with a circumference as large as 100 kilometers (about 62 miles). If funded and built, the FCC would not be finished for decades. Still, scientists at the National MagLab and elsewhere have been researching materials and technologies that could meet the project's demanding specs.
Finding and Testing the Best Materials
Magnets are the backbone of particle accelerators, responsible for focusing particles into tight bundles, steering them through the machine at close to the speed of light and measuring post-collision results. To meet the HiLumi goal of generating more collisions (and more Higgs bosons), much stronger magnets are needed.
"For the scientists, that means physics happens 10 times faster," explained Lance Cooley, an associate director of the National MagLab. Cooley is a manager in the DOE's HiLumi Accelerator Upgrade Project, or AUP, a $220-million effort to build dozens of special magnets called quadrupoles that will focus HiLumi's particle beams.
The superconducting magnets in HiLumi will be 50 percent stronger than current collider magnets, generating fields of up to 12 teslas x Tesla, or T, is a unit of magnetic field strength; a strong refrigerator magnet is .01 tesla, and a typical MRI machine is 1.5 to 3 tesla. The MagLab's strongest persistent magnet has a field of 45 teslas. . (While much stronger than a typical MRI magnet of about 2 teslas, these collider magnets are far less powerful than the research magnets in use at the National MagLab, which can reach up to 100 teslas. However, the MagLab's magnets are engineered differently and serve a different research purpose.) Unlike the wires in LHC's existing magnets, made from niobium-titanium, wires for the new magnet will be drawn from niobium-tin, which performs better in high magnetic fields.
Cooley, a professor of mechanical engineering at the FAMU-FSU College of Engineering who studies particle accelerator technologies, has been in charge of sourcing the 1,800 kilometers (about 1,118 miles) of wire needed just for the focusing magnets the U.S. is contributing to HiLumi. That wire is being fabricated to CERN's specs by Bruker Corporation.
Cooley turned to MagLab physicist Jun Lu to oversee testing of the product. Lu had previously tested niobium-tin wires for the ITER nuclear fusion reactor, another large-scale European science instrument featuring powerful magnets now under construction in southern France. But the CERN project was a new kind of challenge.
"This wire is very different from ITER," said Lu, whose tests make sure those wires perform in the high-field, low-temperature environment that awaits them. "Although it's also niobium-tin, the internal microstructure is different." Niobium-tin is also sensitive to mechanical strain, Lu added, which makes it tricky to evaluate.
Materials scientists Kyle Radcliff (left) and Bob Walsh conduct low-temperature tensile tests to determine the performance and reliability of materials used in cryogenic superconducting magnets.
Photo: Stephen Bilenky
Once the wire passes muster, it is braided into cables at Lawrence Berkeley National Laboratory (LBNL), then wound into coils that are grouped by fours to create a magnet. These coils are then slid into an aluminum shell, which compresses the structure during the cryogenic cool down. Another MagLab expert, materials engineer Bob Walsh, has been testing aluminum samples to see how they hold up to the temperature extremes they are exposed to during fabrication and in use. He measures tensile strength (the force required to rip it apart) and fracture toughness (the strength the material retains after it cracks) by testing samples in liquid helium at temperatures of about -450 degrees Fahrenheit (about -270 degrees Celsius).
His measurements may seem like tiny pieces of data in the grand scheme of the world's biggest particle accelerator. But they are anything but trivial: Project managers sought out Walsh's expertise after the aluminum housing of a test magnet unexpectedly failed.
"It feels good to work for CERN," said Walsh, a MagLab veteran who has also tested materials for the ITER project. "They have done the tests themselves, and they're relying on our expertise to make sure they got the right answer."
Pushing the Limits of Niobium-Tin
While HiLumi's leap from 8- to 12-tesla magnets is ambitious, the proposed FCC is on a totally different scale. "This is a huge endeavor to really understand the underlying physics of the universe," said David Larbalestier, chief materials scientist at the MagLab.
Larbalestier and Cooley represent the National MagLab on the management team of the U.S. Magnet Development Program, under the DOE's Office of High Energy Physics. The program, which also includes partners LBNL, Brookhaven National Laboratory and Fermilab, is exploring longer-term magnet options for the colliders of the future.
The FCC's much larger design calls for much stronger, 16-tesla magnets. (Fermilab scientists are well on their way, having reached 14.1 teslas in 2019). While 10 tons of superconductor will be used for HiLumi, FCC would need 9,000 tons, Cooley said.
Research scientists Shreyas Balachandran (left) and William Starch draw lengths of niobium-tin superconducting wire at the Applied Superconductivity Center at the National High Magnetic Field Laboratory. They are working to increase the amount of current the wire can carry to enable the creation of stronger magnets.
Photo: Stephen Bilenky
The demand for so many strong superconducting magnets means pushing wire technology well beyond current limits. Some researchers have been looking to the new kids on the block, high-temperature superconductors (HTS), as the key ingredient for next-generation wires. These relatively new materials — mere babes compared to old-timer niobium-tin — include compounds based on rare-earth elements and on bismuth, and boast properties untouchable by niobium-tin. Researchers are vigorously studying these compounds, which remain strong candidates for use in FCC magnets. But there are disadvantages.
"There are recent developments in HTS that show a lot of promise. But the problem with HTS is its cost," said MagLab researcher Shreyas Balachandran. "They are currently five to 10 times the cost of niobium alloys. It's really not an option if you need to make kilometers of wire."
Balachandran is betting on another superconductor: that old standby, niobium-tin.
Discovered as a superconductor in the mid 1950s, niobium-tin has been a workhorse in nuclear magnetic resonance (NMR) machines for more than half a century. It has been reliable and predictable, even if its performance as a current carrier peaked a decade ago.
Or so it was believed. Recent research at the National MagLab and elsewhere has demonstrated that niobium-tin has far more promise for next-generation particle accelerators than had been realized.
For decades, niobium-tin fabrication has involved more or less the same recipe. But last year Balachandran and other colleagues at the MagLab tweaked that recipe, with remarkable results. By adding either the element hafnium or zirconium to the mix, they boosted the wire's capacity to carry current by 50 percent. And more electricity means a stronger electromagnet.
"It's a kind of a simple fix," said Larbalestier, corresponding author on the resulting paper. "What is surprising in this case is, in fact, that the refinement is very substantial."
These fractography images show conventional niobium-tin wire (left) and niobium-tin wire with some hafnium (right). The hafnium-containing sample features smaller crystal grains (less than 100 nanometers), which means more grain boundaries in a given area. This feature leads to better performance as a superconductor.
Image: Shreyas Balachandran
The presence of hafnium (or zirconium) changes the structure of the niobium alloy, resulting in smaller niobium-tin crystals and hence more boundaries between these grains in any given area. More boundaries are good because, when in a magnet, they immobilize, or "pin," magnetic flux lines, which boosts superconducting current density.
"The more grain boundaries, the better the conductor will be," Balachandran summarized. "We have shown that there is no intrinsic limit to performance. ... These results have led to the magnet community recognizing that hafnium additions could be the future for niobium-tin."
Mike Sumption, a researcher from Ohio State University, has also made progress experimenting with niobium-tin fabrication techniques. He and his international team have been developing a recipe that features zirconium oxide.
"We're excited to be making good strides on a very practical material," said Sumption during recent experiments at the National MagLab.
Their recipe, however, would require a different production approach, said Balachandran, whereas wire made using his team's hafnium tweak could be fabricated using conventional wire architecture.
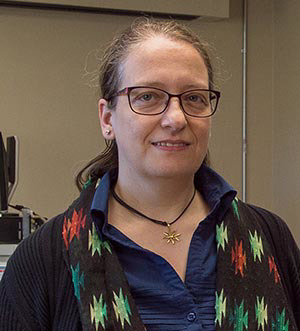
Chiara Tarantini
"We think that the hafnium doping can be implemented in commercial wire relatively easily," said MagLab physicist Chiara Tarantini, a co-author on the paper and principal investigator for this research they are conducting on CERN's behalf. "You don't need to completely change the design of the conductor."
The research team is leveraging MagLab tools and expertise both to understand the feasibility of large-scale industrial manufacturing of the new alloys and to test prototype conductors for superconducting properties. Balachandran said the MagLab offers a uniquely collaborative environment for this fast-paced research and development. "If you have good ideas, you have freedom to explore them," he said. "No one has ever stopped me."

We think that the hafnium doping can be implemented in commercial wire relatively easily. You don't need to completely change the design of the conductor.
- Chiara Tarantini
Similarly, no one has ever stopped the MagLab's young talent from migrating beyond its campus — nor would they want to. A key way the MagLab benefits CERN is by exporting its early-career scientists to other facilities that support the HiLumi and FCC missions. That's what happened to Chris Segal, who did graduate research in mechanical engineering at Florida State University and the MagLab on niobium-tin wires. Segal worked on improving wires fabricated by a method called powder-in-tube, introducing heat treatment techniques that may improve the wires' performance under the stress of high fields.
A postdoctoral position took Segal to CERN, where he learned that there was room to improve HiLumi materials when tested under stress.
"Then I said, 'Well that's interesting, because I have this heat treatment that may be able to help with that.'"
Research into better superconductors won't benefit just physicists and particle colliders: the materials are also used in MRI and NMR machines that help scientists understand the molecular structure of diseases and their effects on the body. Mechanical engineering professor Cooley, though, is in it for the particle physics, and has been since he was a teenager. That's when he met physicist Jim Cronin, who had recently received the Nobel Prize for discovering matter-antimatter asymmetries in K-meson decay.
"Ever since then," said Cooley, a bit starry eyed, "over the last 35 years, I have always been doing something for high-energy physics in some way."
Sure — it's hard for other labs to compete with particles zooming through the subatomic demolition derby that is CERN. But when you're on the pit crew that helps build the machine where it all happens, you're not competing anymore: You're on the team.
Story by Kristen Coyne