In a brutish, one-of-a-kind oven at the National High Magnetic Field Laboratory, scientists have been creating volcanic conditions that may lead the evolution into the next great Age of Magnets.
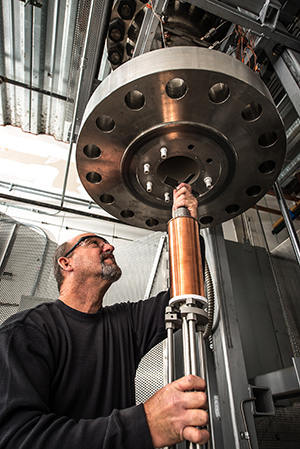
A scientist from the UK doing research using the lab's 45 tesla hybrid magnet.
Image credit: Dave Barfield
The oven's high pressure (up to 100 bar, or 1,500 pounds per square inch) and temperature (up to 1,650 degrees Fahrenheit, or 900 degrees Celsius) create a magma-like environment that is perfect for making magnet coils made from a promising kind of superconductor.
For several years, scientists and technicians at the MagLab's Applied Superconductivity Center (ASC) have been tweaking the 2-ton instrument, built per the lab's specifications by Deltech Inc. This exercise in vulcanology has allowed them to tap into the powers of a special kind of ceramic superconductor known as BiSCCO (rhymes with disco). Learning lots of lessons along the way, they have tested increasingly more powerful (ahem) "BiSCCO-tech" magnets. Most recently, they cooked a 3.5-tesla coil (tesla is a unit of magnetic field strength; a typical hospital MRI is 2 or 3 teslas). When placed inside a 31-tesla resistive magnet, the smaller coil helped generate an overall field of 34.5 teslas that had engineers boogying across the floor. (OK, we're just kidding about that last part.) That test validated the capability of BiSCCO at high magnetic fields.
In fact, the ASC team has made so much progress that the U.S. Department of Energy has funded an even larger version of the furnace, to be designed, built and operated at the ASC.
Its mission: Demonstrate accelerator magnet technology beyond CERN's Large Hadron Collider and set new world records for precision laboratory magnets.
First, a few words about superconductors
Superconductors are special materials that, unlike copper, carry electricity without resistance. Most superconducting magnets today, such as MRI magnets, only work when they are extremely cold, a state that requires expensive, complicated liquid helium cooling systems (liquid helium is −269 degrees Celsius or –452 degrees Fahrenheit!)
Some more recently discovered materials become superconducting in relatively warmer environments achievable with cheaper, abundant liquid nitrogen (–176 degrees Celsius, or -285 degrees Fahrenheit) — a significant practical advantage. These so-called "high-temperature superconductors" (HTS) include bismuth strontium calcium copper oxide, or BiSCCO for short. For magnets, that extra headroom in temperature is key because very high fields can be reached when HTS are operated in liquid helium.
Scientists have been developing HTS materials since they were discovered in the 1980s. They became available in long-length wires and tapes in the early 2000s, and the first HTS magnets followed about a decade later. A key requirement for building magnets is a high capacity for carrying current in the superconductor. For a while Bi-2212 — one of several variants of BiSCCO — was considered less promising because it couldn't carry as much current as other HTS, including rare-earth barium copper oxide (REBCO).
"In the early stages, the current density wasn't that great, and REBCO supporters said, 'Why are you bothering?'" recalled MagLab Chief Materials Scientist David Larbalestier, who is overseeing the new furnace project. "And we said, 'Because we think we can understand it and do better.'"
And they did: By subjecting Bi-2212 to high pressure and high temperatures, they unlocked the superconductor's enormous potential.
Troubling bubbles
To make a Bi-2212 magnet coil, scientists begin with the compound in powder form, cram it into a silver tube, then draw it down into thinner and thinner filaments until it's one-fifth the diameter of a human hair. Hundreds of these filaments get bundled into a silver-clad wire about a millimeter wide that gets wound into a coil — the heart of a superconducting magnet.
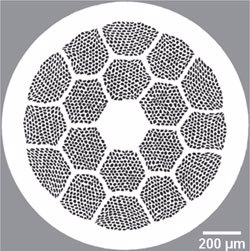
Cross section of Bi-2212 wire.
The image at left illustrates the result of all this drawing and bundling: a wire cross section that appears to be packed solid, (the black areas are the Bi-2212). In fact, about a third of each black area is empty. More precisely, the empty portion is filled with gas. These pockets are dead-ends for electricity, and for years have kept Bi-2212 from reaching its full current-carrying potential. ASC engineers knew that getting rid of those bubbles would get rid of the problem.
"Anybody who has lived in snow country knows that if you have 10 inches of snow, you get about 1 inch of water," explained ASC engineer Eric Hellstrom, an expert on BiSCCO. "So you get all this empty space between the ice crystals. We have exactly the same thing with BiSCCO."
To remove empty space out of snow and make a good strong snowball, you squeeze it between your hands and partly melt it. Similarly, to remove the extra space out of Bi-2212 wire, you squeeze the heck out of it when it is molten and as it cools.
The ASC team came up with a technique to do just that called overpressure processing (OP), and it works like this: Pop your Bi-2212 coil into the furnace, put the 5.25-inch thick lid over the container, bolt the thing shut with numerous 15-inch, 17-pound bolts and a pneumatic torque wrench, crank the temperature way up and pump in a special mixture of argon and oxygen gas at a pressure 50 times greater than in a car tire.
The high temperature melts the Bi-2212 powder in the wires, and the high pressure counter-acts the troublesome bubbles. The conditions are like those below a volcano, where heat and pressure melt rock and dissolve gasses into the magma.
With the obstructions removed, the coil's electrical capacity is seven times better than it would have been without this process. The treatment also makes the material stronger.
The round wire shape of a BiSCCO coil is also a big bonus. While HTS magnets can be made with superconducting tape (see the MagLab's world-record 32 tesla all-superconducting magnet), round wire has many advantages. Almost all of today's superconducting magnets are made of round wires (mostly using low-temperature superconductors niobium-tin and niobium-titanium). So using Bi-2212 means magnet makers don't need to redesign coils.
Yet another BiSCCO advantage: It's electromagnetically isotropic. "That's a very important aspect," explained ASC researcher Ulf Trociewitz, who is directing the effort to develop Bi-2212 magnets. "You can actually place this type of conductor in any position inside the magnet without changing its properties."
Finally, BiSSCO and other HTS materials can make more powerful magnets because niobium-based magnets max out at about 23 teslas.
Boon for biomedical and physics research
That's why scientists are looking to HTS superconductors like REBCO and BiSCCO for future superconducting magnets.
Physicists and engineers have been planning the successor to the Large Hadron Collider, an instrument that relies on thousands of superconducting magnets to accelerate particles. They envision a collider seven times more powerful than the current one, requiring magnets two to three times stronger than the collider's existing magnets. In collaboration with Lawrence Berkeley National Laboratory in California, ASC has been developing and processing Bi-2212 coils in its OP furnace that may evolve into the dipole magnets that help make CERN's dreamed-of upgrades a reality.
Over in the biology and chemistry departments, scientists are just as excited. They are eager to explore the new worlds that will open up to them in precision superconducting magnets of 40 teslas or even higher. A 40-T nuclear magnetic resonance (NMR) magnet would mean much higher resolution and detail for scientists studying complex proteins, as well as better image resolution and data for research in drug development, biochemistry and in-cell, pre-clinical, and human studies.
"No single factor can increase the quality of NMR spectra from liquids or solids as much as an increase in the operating magnetic field," said Lucio Frydman, chief scientist for chemistry and biology at the National MagLab. "Throughout NMR's history, each time a quantitative jump in the field became available, high-impact biomolecular, biophysical and material sciences breakthroughs quickly followed. About a decade ago, such jumps came to a standstill — until now."
By Kristen Coyne