Contact: Ryan Baumbach or Kristin Roberts
TALLAHASSEE, Fla. — Exploring an area overlooked by other scientists, physicists at the National MagLab have discovered that a class of materials called “1-2-20s” (one-two-twenties) have very promising thermoelectric properties. The finding opens the floodgates for further research into these fascinating materials, which offer scientists ample moving parts that can be swapped out to improve their efficiency. The study was published today in Science Advances.
Thermoelectric devices can produce electricity if there is a temperature difference between the two ends. They can also do the opposite: use electricity to absorb or release heat. This property has lots of actual and potential applications, from compressor-free refrigeration to power generation in space to recouping all the energy wasted by car engines (about 40 percent) that escapes through heat.
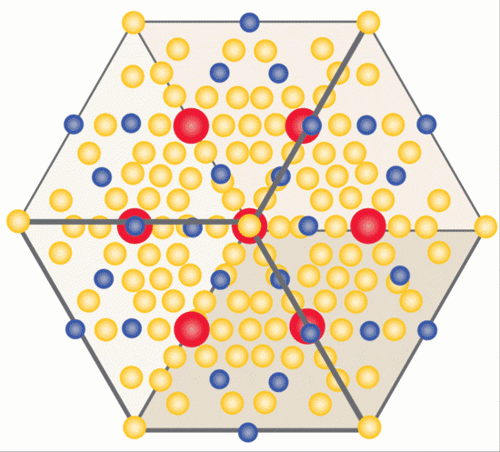
"It's not free energy," said MagLab physicist Ryan Baumbach, corresponding author on the paper, "but it’s the next best thing."
Most materials have very little thermoelectric effect. That's because the transfer of electricity across a material and the transfer of heat usually go hand in hand. To have a good thermoelectric, these two properties need to be decoupled. You want to encourage the movement of electrons for the current, while at the same time discouraging thermal transport, because the heat gradient needs to be preserved to maintain the flow of electricity.
In general, nature wants to keep heat and electrical conductivity linked. But about two years ago, Baumbach suggested that Kaya Wei, the MagLab's Jack Crow postdoctoral fellow and a member of Baumbach's research group, study a "1-2-20" material that seemed like a good candidate for thermoelectricity.
The specific material Baumbach proposed featured three basic ingredients in a “1-2-20” ratio: the element ytterbium; a transition metal (either cobalt, rhodium or iridium); and the element zinc. Baumbach had a hunch this compound had what it takes, if manipulated properly in his lab, to thumb its nose at nature and unlink thermal conductivity from heat conductivity. He suggested Wei first comb through the science literature to see what other scientists had learned about it before launching her investigations.
But, to both their astonishment and delight, Wei found almost nothing.
“I was completely baffled that no one had looked at them yet,” said Baumbach. “It just seems really obvious.”
So Wei got cracking. Using high-temperature furnaces in Baumbach's lab, she synthesized the compound in crystal form and subjected the samples to a gauntlet of measurements. The results confirmed that, at low temperatures, the material was in fact a promising thermoelectric. Bingo.
Then it was time to start playing around with the variables to see what else they could discover. “Different compositions promote quite different physical properties,” explained Wei, the paper’s lead author.
Building a better thermoelectric
The researchers wanted to make a material as thermoelectrically optimized as they could, a property represented by a parameter called the thermoelectric figure of merit (or ZT). To do that, they needed to tweak their crystal to: 1. Maximize its electrical conductivity; 2. Minimize its heat conductivity; and 3. Develop a large voltage when a small temperature gradient is applied (i.e., when one end is slightly warmer than the other), a property measured by a value called the Seebeck coefficient.
The first goal was the easiest: The material was already a good conductor in large part thanks to the zinc and transition metal.
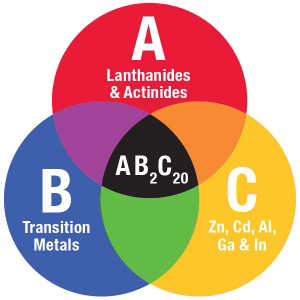
The class of materials known as “1-2-20s” feature three basic ingredients in a “1-2-20” ratio.
Image credit: Caroline McNiel
The other goals were more complicated. To achieve the second, the scientists needed to sabotage the phonons that are largely responsible for carrying heat. Phonons are vibrations that propagate through a material’s three-dimensional atomic lattice: In this way, energy absorbed by an atom can ripple, atom to atom, across the entire material.
Luckily, inherent to the very structure of 1-2-20 materials was a way to throw up massive phonon roadblocks.
The “20” part of each of the unit cells in the crystal Wei made was a cage-like structure comprising 20 zinc atoms — a cage big enough to house the “1” part of the unit cell, an ytterbium atom. And just as an unruly criminal jostles the bars of his prison, that heavy, dense ytterbium atom rattles around in its cage, creating quantum mayhem. The rattling interferes with the ability of phonons to dissipate heat through the material, creating a massive phonon traffic jam. The crystal’s very large unit cell bolsters this effect. It’s a bit like interfering with the trajectory of a clay pigeon with a well-aimed bullet: The phonons are scattered around every which way.
The ytterbium lends another important ingredient to the compound’s thermoelectric success. It contains a kind of electron called an “f electron.” Without getting too quantum mechanical, f electrons tend to stay close enough to the nucleus to maintain a magnetic character. In ytterbium and some other special cases, however, f electrons also behave like a tentative toddler meeting someone new: They vacillate between clutching tight to their nucleus mom and venturing out toward neighboring atoms.
“The ytterbium f electrons are special because they have a duality between being localized and delocalized,” Baumbach explained. “This helps account for the material’s large Seebeck coefficient.”
Next steps
Now that they have discovered and better understood this recipe for thermoelectricity, Baumbach and Wei are exploring further. The ZT values of the compounds they tested peak at very low temperatures — around -400 degrees Fahrenheit or -240 degrees Celsius. This would be useful in space or for other low- temperature applications only. But by experimenting with the specific ingredients in their 1-2-20s, the scientists say they can achieve different results.
“There are so many chemical variants for the 1-2-20 family of compounds,” Wei said. “It’s not just that you would change 100 percent of one element or another, but you could do chemical substitution. And our hope is, in doing that, we’ll be able to move around the temperature where the ZT value peaks and find materials for different applications.”
They also recently discovered that putting their compounds in one of the MagLab’s high-field magnets increased the ZT, results they plan to publish soon.
Although pleased with their success, Baumbach and Wei seem even more excited to have opened a whole new can of worms with their science that will attract flocks of other researchers. The more, they say, the merrier.
“These guys are just a few examples of a really big family of materials,” Baumbach said. “We think that this work will stimulate a lot of interest from groups outside of our own.”
Story by Kristen Coyne