Thanks to the Nobel Committee for Physics, topological materials are now top news. This esoteric area of research is making headlines worldwide, and scientists are struggling to make this stuff make sense to the rest of us.
But at the National MagLab, it's old news: Researchers working here have been experimenting with these materials, making exciting discoveries and publishing their findings for more than a decade.
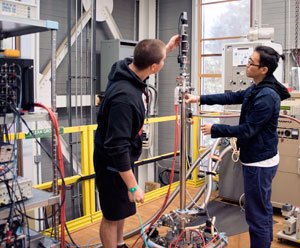
Petr Stepanov (left) and Jiawei Yang from the University of California Riverside research topological matter at the MagLab.
In fact, high-field magnets are among the most important tools available to scientists for finding topological matter and probing the exciting and promising behaviors that occur within them. Thanks to its world-record magnets and expertise in experimental condensed matter physics (science-speak for the study of solids and liquids), the National MagLab is at the forefront of this research.
"High magnetic fields are indispensable in figuring out how topological materials work," said physicist Tim Murphy, director of the MagLab's DC Field Facility, where about 20 percent of the experiments are devoted to the increasingly hot topic.
Nobel news
In case you missed it earlier this week, the Nobel Prize in Physics was awarded to David Thouless, Duncan Haldane and Michael Kosterlitz "for theoretical discoveries of topological phase transitions and topological phases of matter." The announcement left a lot of people scratching their heads over "topological," but we'll get to that in a minute.
In the decades since the three theorists published their revolutionary work, experimentalists at the National MagLab and elsewhere have been busy — and never more than in recent years. They are finding more materials that belong to this category of matter, discovering it in different shapes, observing its strange behaviors under extreme conditions (like cold and high pressure), and building a base of knowledge that could pave the way to inventions like quantum computers that could profoundly change our world.
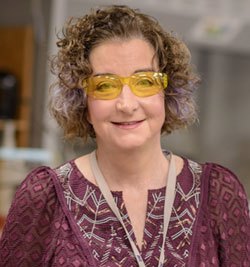
MagLab Chief Scientist Laura Greene says research in topological materials could be "transformative."
"The applications from these new families of correlated electron materials could be transformative," said MagLab Chief Scientist Laura Greene.
During the Nobel announcement on Tuesday, Haldane expressed astonishment over how far experimental physicists have come in proving, then expanding upon the phenomena predicted by him, Thouless and Kosterlitz in the 1970s and 1980s.
"That was a long time ago, but it's only now that a lot of tremendous new discoveries which are based on this original work, and have extended it in many ways, are now happening," said Haldane, a Princeton University physicist. "It turns out that a lot of materials that people had looked at for many years did actually have these topological properties, but had just never been seen because people hadn't looked for them."
Topo what?
In condensed matter physics, "topological" is cropping up in permutations that twist both tongue and brain: topological matter, topological insulators, topological phase transitions, topologically protected states, topological entanglement entropy, topological nodal-line fermions, topological berry phases, topological metamorphosis and topological two-dimensional metallic surface states – to name a few.
A look at the research coming out of the MagLab underscores the scope of this boom. In 2015, 35 of the lab's research reports related to topological materials, up 75 percent from just five years earlier.
All of which begs the question: What the heck is topology?
In a nutshell, it's a mathematical abstraction that, when cleverly applied to physics, led the theorists to predict the existence of highly unusual states (unlike anything we see in the "normal" world around us) in certain materials under certain conditions.
The concepts aren't easy to grasp. But the good news is, you don't need a PhD in math to appreciate the impact of the theorists' work: That special materials (topological materials) exist; that they can exhibit unusual behaviors (topological states) beyond the everyday norm; that those states can teach us new things about the physics underlying our world; and that they could one day be tapped for next-generation electronics and other useful applications.
At the end of the day, the study of topological materials, like much of condensed matter physics, is all about the ways electrons behave, and how that can be harnessed to make stuff work.
"The world that we live in," said physicist Murphy, "is determined by the electron — the material that it's in and how it interacts with other electrons."
Some electron behaviors are conventional (the electricity powering your computer) and some, like topological states, are exotic.
The power of topological states
Scientists are uncovering different kinds of topological states. One is the quantum Hall effect (another Nobel Prize-winning advance), in which electrons in some two-dimensional materials change their ability to conduct electricity in discrete steps, rather than gradually, as the magnetic field is increased.
A compelling characteristic of topological materials is that they exist in a kind of “locked” or “protected” state. This keeps them behaving consistently and predictably, even under stress. It’s largely this robustness that makes them promising as materials for real-world applications.
To MagLab physicist Ryan Baumbach, this rigidness brings to mind cars on a divided freeway.
"If you're in the right-hand lane, you're definitely going in one direction, and if you're on the left-hand side, you're definitely going in the other direction: Their phases are locked,” said Baumbach, whose research group studies topological states of matter. "That's part of the magic of what happens in a topological state. You can throw in some potholes, but they're still going to keep going the same way."
Experimentalists get to work
After the theorists recognized in Stockholm this week cracked the mystery of topological behavior, the experimentalists got cracking. They put different materials inside magnets and other instruments (a technique called angle-resolved photoemission spectroscopy has also been useful to scientists) to see if they could observe some of these predicted states.
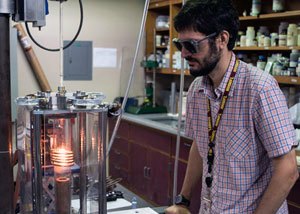
Physicist Ryan Baumbach says topological materials research is still in its infancy.
"High magnetic fields can provide direct evidence of whether a material of interest in fact exhibits topological effects or not, based upon the particular magnetic fields at which the conductivity oscillates," explained MagLab Director Greg Boebinger. "In addition, high magnetic fields often drive transitions between different states of matter in topological systems by changing the ratio of magnetic field strength to the number of electrons and seeing sudden jumps in the conductivity at particular values of the magnetic field."
About a dozen years ago, scientists began finding some two-dimensional materials of interest to this year's laureates, starting with the isolation of graphene in 2004. A one-atom thick layer of carbon, it revealed amazing properties (strength, conductivity, flexibility, thinness and transparency) that promised a slew of practical applications, sparked a spate of experimentation and resulted in a Nobel Prize. In 2007, research at the MagLab demonstrated that graphene shows topological behavior even at room temperature.
Another exciting development in two-dimensional materials occurred at the MagLab in 2013 during experiments in the lab's world-record 45 tesla hybrid magnet (45 T). When the scientists saw a long-predicted energy pattern known as the Hofstadter butterfly in graphene on hexagonal boron nitride, they were observing a topological phenomenon. Their discovery was published in the prominent journal Nature.
Scientists also discovered materials not specifically foreseen by the Nobel trio called topological insulators: While remaining an insulator on the inside, they conduct electricity on the surface. In experiments conducted in the MagLab in 2012, scientists made significant discoveries about the topological insulator CaMnBi2, followed a few years later with findings on BiSbTeSe2. More recently, scientists working in the MagLab's 45 T and elsewhere have been studying samarium hexaboride (SmB6), a different class of topological insulator that may one day be used in quantum computers. And earlier this year, MIT researchers working at the MagLab with a topological insulator fashioned from gadolinium, platinum and bismuth discovered topological properties combined with intrinsic magnetism — findings that could pave a path to spin-based electronics.
So as scientists fabricate more topological compounds and expose them to environments in which they reveal more exotic behaviors that compel them to coin more topological tongue twisters to describe them … what's next?
Just as laureate Haldane had little inkling of the long-term transformative effects of his theories, we can only guess where recent findings on topological states will lead.
"I really think the things that we've found so far are kind of beacons," said physicist Baumbach, "things that are telling us this is the right path to go down — but they're not the thing yet. It's something marking the path, but it's not the end of the path.
"It's just in its infancy," Baumbach added. "There's a lot of potential for growth in terms of new materials and electrons behaving in new ways."
By Kristen Coyne
Lead illustration by Caroline McNiel. Greene photo by Dave Barfield. Other photos by Stephen Bilenky.