Super ... to a point
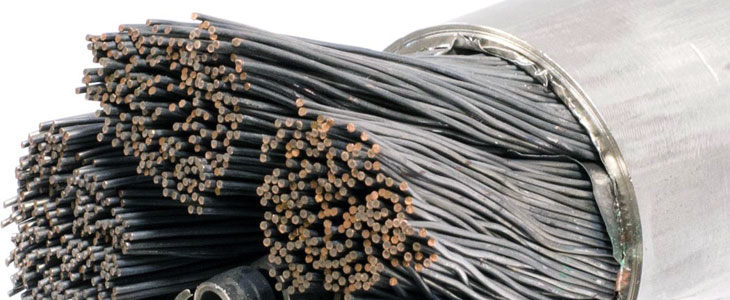
Wires of superconducting niobium-3 tin, a "low-temperature" superconductor, after partial removal of stainless steel jacket to reveal in the internal components.
Image by Carlos (Charlie) Sanabria / National MagLab
Electrical wire is made of copper because it’s a good conductor of current. But it’s far from perfect: Those current-carrying electrons careen through it like pinballs, bashing into each other and wasting energy in the process. In fact, 6 percent of electricity generated in the U.S. is lost between the power plant and its destination in your home or business because of this inefficiency.
That's why superconductors are so exciting: They are 100 percent efficient because current zooms through them with zero energy loss: The pinball machine turns into a super highway! This is great for the electric bill, one reason (but not the main reason … more on that in a minute!) why we make and use lots of superconducting magnets at the National MagLab.
But there's a rub: Conventional superconductors (like niobium-tin and niobium-titanium) operate only at temperatures so low that you need expensive (and non-renewable) liquid helium. to create them. That puts a limit on how much you can do with these "low-temperature" superconductors. Yes, they are super — but only to a point … very low on the thermometer!
Warming trends
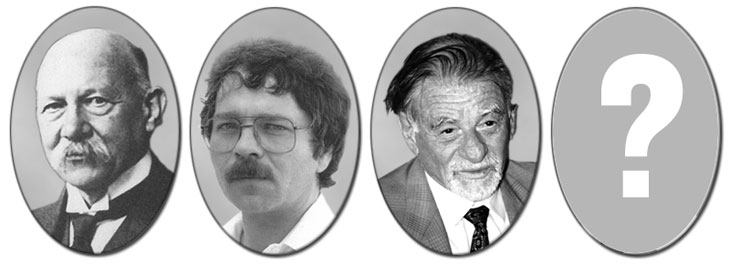
Superconductivity pioneers (from left to right) Heike Kamerlingh Onnes, J. Georg Bednorz and K. Alex Müller. Who will be next?
The history of superconductivity dates to 1911, when Heike Kamerlingh Onnes discovered the property in mercury that had been cooled to −269° Celsius (about −452° Fahrenheit).
But the field took a giant leap in the mid 1980s. That’s when J. Georg Bednorz and K. Alex Müller, playing with a class of materials overlooked by other scientists, discovered a copper oxide compound able to superconduct at -238° C.
While still pretty chilly, that’s far warmer than liquid helium temperatures. So it became known as the first “high-temperature superconductor” ever discovered. The Nobel-prize winning news hinted that scientists might eventually find superconductors that work in warmer (albeit still chilly!) environments attainable with liquid nitrogen (-196° C, or -320° F), which is cheap and plentiful.
You can think of these materials (referred to also as HTS or high-Tc superconductors) as super duper conductors. After their discovery, all heck broke loose as scientists scrambled to find materials that superconduct at ever warmer temperatures. Many dreamed of transformative discoveries that could dramatically cut the cost of transmitting electricity.
Stronger Magnets for Bigger Discoveries
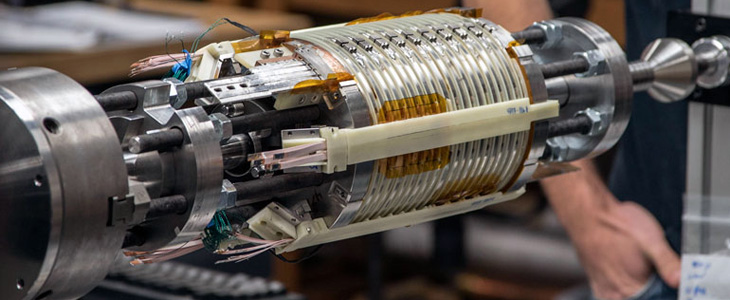
The Natonal MagLab's 32-tesla all-superconducting magnet, featuring YBCO, under development.
Image: Stephen Bilenky / National MagLab
One HTS application is already a reality: stronger research magnets, which scientists use to study materials, disease, complex molecules like oil and proteins, among many other areas.
As it turns out, not only do HTS materials work at warmer temperatures: They also perform in very high magnetic fields. Their low-temp counterparts stop working at around 23 teslas (tesla is a unit of magnetic field strength), so you can’t use them to make magnets stronger than that. But HTS materials can work at more than twice that field, and likely even higher. For proof, look no farther than the National MagLab, where a superconducting magnet with a world-record field of 32 teslas is slated to come online in 2017.
Scientists continue to hunt for new materials that superconduct under various conditions (in addition to lowering the thermostat, increasing pressure can change a material into a superconducting state). It’s hard to keep up with the latest research occurring at the MagLab’s Applied Superconductivity Center (ASC) and elsewhere. But here is a primer on some of today’s most promising high-temperature superconductors, based on what we currently know.
Cuprates
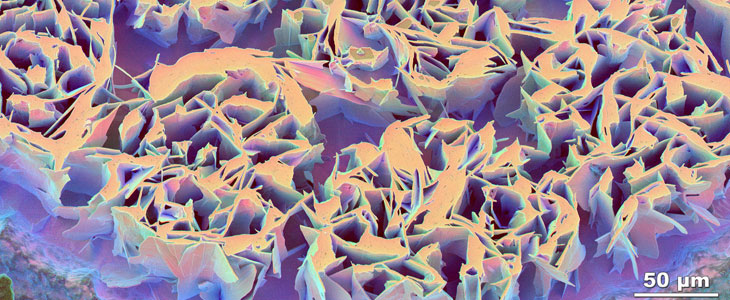
High density Bi-2212 filament macrostructure produced by the over-pressure (OP) technique developed at the Applied Superconductivity Center.
Image credit: Peter Lee
Also called ceramics, cuprates are materials made up of oxygen and copper layers first discovered to be superconducting (as mentioned above) by Bednorz and Muller in the mid 1980s. Because ceramics are brittle, scientists and engineers had to figure out how to manufacture them into wires, tapes or other practical shapes — easier for some compounds than others.
First came BSCCO, shorthand for bismuth strontium calcium copper oxide — or "bisco" (rhymes with Crisco). Within a few years of the Bednorz/Muller discovery, engineers were making wire of it, which is now used in some very high-tech transmission cable, transformers, motors and generators. And, of course, magnets!
There are different types of BSCCO, depending on its exact chemical composition, including Bi-2223 and Bi-2212. The MagLab is using the latter to build a novel research magnet dubbed the Platypus, a high-field (24-tesla) instrument for nuclear magnetic resonance research. Elsewhere, scientists are working with Bi-2223 to build current leads for research magnets. Both of these forms of BSCCO superconduct well above liquid nitrogen temperatures. Of all classes of high-temperature superconductors, cuprates continue to boast the highest transition temperature — the point below which they are superconducting.
ReBCO/YBCO
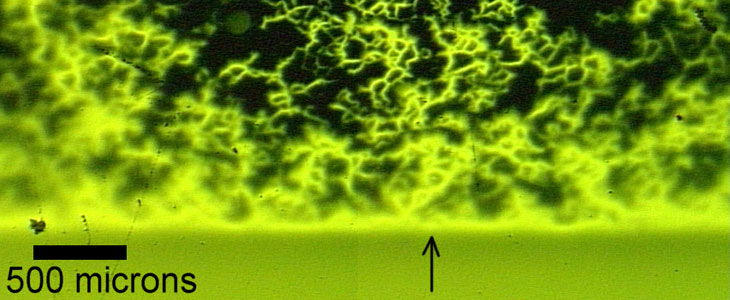
Magneto-optical image of a 3.3 mm wide YBCO on RABiTS coated conductor.
Image credit: ASC
A cousin of BSCCO is ReBCO, another cuprate. In this high-Tc superconductor, the barium, copper and oxygen found in BSCCO is joined by one of the rare earth elements (hence the "Re"). One variation is YBCO, the Y standing for the rare earth element yttrium. In collaboration with industry partners, MagLab scientists and engineers have done extensive research and development on ReBCO and YBCO, which has a transition temperature of about -181 degrees Celsius. MagLab engineers developed a windable tape out of YBCO and used it in a magnet that broke the world record in 2017 for an HTS coil operating inside a high-field resistive magnet.
YBCO is used in another novel MagLab magnet. Projected to crush the existing world record for the strongest superconducting magnet when completed in 2017, the 32-tesla magnet will feature about 6 miles of YBCO tape. Like the Platypus, it will combine high-temperature and low-temperature superconducting magnet coils. YBCO is also currently in use in numerous applications, including power transmission cables, motors, generators, bearings and microwave filters for cell phone towers.
Borides
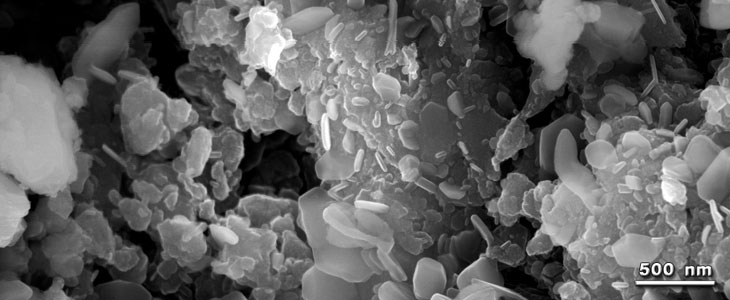
C-doped MgB2 Nanocrystals.
Image credit: Peter Lee / National MagLab
Magnesium diboride (MgB2) was discovered to be a high Tc superconductor in 2001. It actually falls somewhere between a low-temperature and high-temperature superconductor. It appears to work, at least in part, like a low-temperature superconductor: via a phenomenon known as Cooper pairs. (By contrast, scientists don’t yet understand the mechanism behind most high-temperature superconductors — but they know it’s not Cooper pairs!) However, MgB2 remains superconducting at higher temperatures than its low-temp cousins can manage (its critical temperature is -234 degrees C).
One of MgB2's biggest advantages is its low price tag. Scientists at CERN have demonstrated that, when cold, it’s an outstanding conductor, findings that suggest it could be used in long-distance power transportation.
"MgB2 is cheap, abundant and lightweight," said engineering professor Eric Hellstrom, who studies high Tc superconductors at the ASC. "For that combination of properties, there may be a special niche of potential applications."
Iron-based superconductors
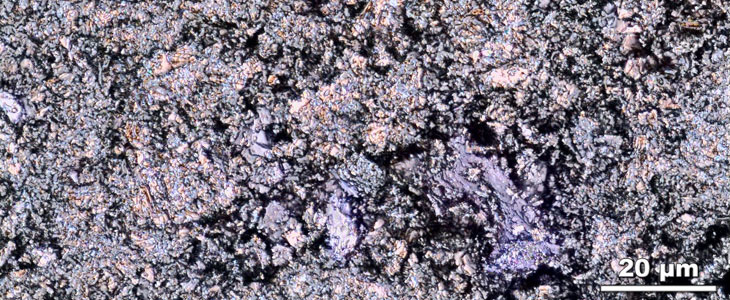
Fractured surface from LaFeAs-OF sample imaged using a laser scanning confocal image.
Image credit: ASC
Also called ferropnictides, iron-based superconductors (IBSCs) are iron-containing compounds that are relative newcomers to the high Tc family. They feature layers of iron and a pnictide, such as arsenic or phosphorus. Their discovery in 2008 came as a surprise to many, because iron was seen as incompatible with superconductivity. Turns out it isn’t, and that IBSCs have properties that are helpful for turning them into current-carrying wires. They also have a respectable transition temperature (although not as high as the cuprates).
Scientists have been aggressively studying IBSCs. One question driving their work is the same question asked by scientists studying any other high-temperature superconductor: How do they work? While scientists have known for decades how low-temperature superconductors work (this introduction to superconductivity explains it), they don’t yet understand what’s behind high-temperature superconductors. Is there a single explanation, or does each family of HTS material work for different reasons?
Physicists everywhere are working to unravel these mysteries. Their work is paving the way for stronger research magnets and, one day, could revolutionize how electricity is transmitted to our homes, factories and businesses.
Story by Kristen Coyne