Heike Kamerlingh Onnes never would have discovered superconductivity back in 1911 if he hadn’t first learned to make liquid helium, which created the frigid temperatures the phenomenon required.
In the 1950s, scientists wouldn't have discerned the double helix hiding inside DNA if the X-ray diffractometer hadn't first been invented.
And those gravitational waves that were all over the news in 2016? You can thank the $1 billion (and counting) Laser Interferometer Gravitational-Wave Observatory for making that science milestone possible.
Some of science's biggest blockbusters remain hidden until the right tool comes along. That's why scientists dream of stronger, faster, further and, in the case of magnetic fields, higher.
Physicists exploring electrons, chemists decoding complex molecules and biologists scanning brain cells are clamoring for next-generation instruments. The U.S. National Academy of Sciences has advocated for them for years.
The next big magnets are overdue: The world's strongest continuous-field magnet (the 45-tesla hybrid at the National High Magnetic Field Laboratory) has held the title for two decades. A few slightly stronger instruments are slated to come online in coming years, but those are merely hops forward. Scientists want leaps.
They may soon get them. Thanks to recent advances in magnet technology, strategic government funding and the nudge of international competition, the momentum for higher fields may have reached a critical point.
Scientists have been dreaming of them. And they are ready.
New Peaks in Physics
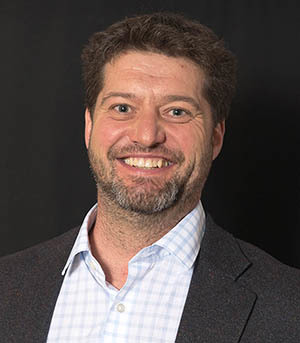
Ross McDonald
Pulsed magnets are the most powerful kind of magnet (although, unlike continuous-field magnets, they can only operate for a fraction of a second). The strongest of them all, located at the National MagLab, reaches 100 teslas — for about 25 milliseconds at a time. Physicist Ross McDonald wants to see it climb higher.
McDonald, deputy director of the lab's Pulsed Field Facility, co-authored a paper in the prestigious journal Science this year that reported a new phenomenon in a type of high-temperature superconductor (HTS) that could be observed only with the help of very high fields. Eighty teslas were needed to suppress superconductivity in the material, which revealed a different conducting behavior new to science.

We've clearly identified an area of physics that we don't understand and where magnetic field is having a profound role.
- Ross McDonald
"We've clearly identified an area of physics that we don't understand and where magnetic field is having a profound role," McDonald said. "And we need these extreme fields of 100 teslas or more to look at this."
But magnets have not kept pace. The most robust HTS materials keep superconducting even in very high magnetic fields — higher than the strongest magnets available today — making it impossible to probe their "normal" conducting states. Yet such experiments are critical for understanding high-temperature superconductivity, one of the hottest areas in physics.
That's why the National MagLab is brainstorming magnet designs that could boost millisecond-duration pulsed fields significantly.
"Given the resources, our designs for magnets exceeding 130 teslas could be a reality within the decade," McDonald said.
To physicist Tim Murphy, director of the National MagLab's DC Field Facility, findings like McDonald's are tantalizing indications of what awaits when the continuous-field magnets he oversees make their next big leap past 45 teslas.
"At pulsed fields, you see hints of physics that you need more time, and a quieter environment, to study," said Murphy. "And that's what a 60-tesla DC magnet can provide."
That's the lab's longer-term target. To get there, lab leaders plan to first design and build a 40-tesla magnet featuring high-temperature superconductors (see The Long Winding Road).
Discovering New Tipping Points
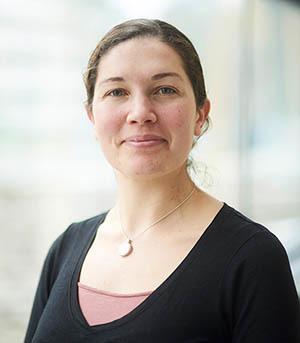
Higher fields could help physicists like Elizabeth Green prove new states of matter.
For Elizabeth Green, those high fields can't come soon enough. A physicist at the Dresden High Magnetic Field Laboratory, Green is eager to put samples of lithium copper vanadate, a compound that may have electronic properties similar to liquid crystals, into continuous fields of 50-plus teslas. At that field strength, she dreams of studying a magnetic state that might be used to develop new electronic devices.
"That would allow us to further probe and, with any luck, definitively prove the existence of the spin-nematic state in condensed matter systems," explained Green.
Physicists like to expose materials to extreme pressures, temperatures and magnetic fields to see what happens to them. Of these environments, magnetic field is particularly cool because you can point it in a specific direction, explained physicist James Analytis.
That makes it really useful for probing anisotropic materials, which behave differently in a magnetic field depending on their orientation, said Analytis, an assistant professor at the University of California, Berkeley. So while the electrons in a material may behave like free electrons in one orientation, he continued, "if you tilt it and point it in another direction, you might find it behaves like a frozen particle, or a strongly interacting particle."
That's the kind of powerful behavior that could one day be engineered into a switch. The stronger their magnets, the more scientists can leverage this vector advantage.
Then there's the matter of scale. Scientists already have a big temperature scale to play with, from near absolute zero to thousands of degrees Celsius. Making just the right tweak can cause quantum systems to break: At 0 degrees Celsius, for example, water turns to ice.
Giving scientists access to a wider range of magnetic fields, Analytis said, could help them find similar tipping points that, by overcoming a system’s internal energy scale, could trigger a sudden change in behavior.
"Sometimes," Analytis said, "in order to understand that physics, you need to see how it breaks up."
Higher Fields For Health
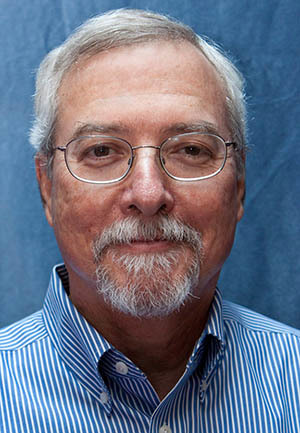
Tom Mareci
Researchers in the health sciences use different kinds of magnets than physicists. Theirs are built with superconductors. For nuclear magnetic resonance (NMR) or magnetic resonance imaging (MRI) experiments, these scientists need fields that are both strong and uniform, which superconductors provide.
MRI machines identify hydrogen in the body, and sometimes other atoms, to create an image of different tissues that reveals tumors and other structures. NMR instruments work similarly, but examine specific tissue samples or molecules rather than whole bodies. The technique pinpoints the location and orientation of atoms so scientists can better understand, say, a protein’s structure or function.
Stronger fields, scientists say, will help them see all this in greater detail as well as identify biologically important atoms that, until now, have escaped the reach of magnetic resonance.
Scientists are proposing a 20-tesla instrument for human brain imaging and chemical detection — almost seven times stronger than a typical hospital MRI and twice as powerful as the strongest MRIs used in human research. Its resolution would be 10 times what can be achieved today, said Thomas Mareci, professor of biochemistry and molecular biology at the University of Florida. In a square millimeter of brain tissue, he said, that's the difference between seeing a lump representing tens of thousands of fibers and being able to identify each individual fiber.
"We want to understand how the brain develops that circuitry, how it might actually grow new circuits, and how it might be affected by an injury," Mareci said. "The only way to understand that without taking the brain apart is to look at it with high magnetic fields."

We want to understand how the brain develops that circuitry, how it might actually grow new circuits.
- Tom Mareci
Thomas Budinger, a physician and physicist at the Lawrence Berkeley National Laboratory, is among those building a compelling case for a 20-tesla MRI. The instrument would probe questions that cannot be answered with other instruments, he said, including how neuron architecture is related to autism, obsessive compulsive disorders, schizophrenia, aging and chronic brain trauma. It could also identify small molecules associated with addiction, violence and depression.
Budinger, who is also a professor at the University of California, Berkeley, where he has taught bioengineering, electrical engineering and radiology, said the ability to watch sodium cross cell membranes could teach scientists about bipolar disorder.
"We could understand the sodium-potassium balance in the brain relative to mental states," Budinger said.
A 20-tesla MRI could also shed light on another problem Budinger has worked on for decades: why some brain tumors resist treatment.
"Since 1976 I have worked with the problem of treatment of this with neurosurgeons and we have made zero progress," said Budinger, "except to give patients quality of life in their last few years from diagnosis."
Studies have shown, he said, that tumor cells that metastasize produce lactate, unlike tumors that respond to therapy. With a strong enough MRI magnet, he continued, scientists could discern the difference and develop methods to measure and even modify the lactate-producing tissue, potentially revolutionizing cancer treatment.
Scoping Cellular Secrets
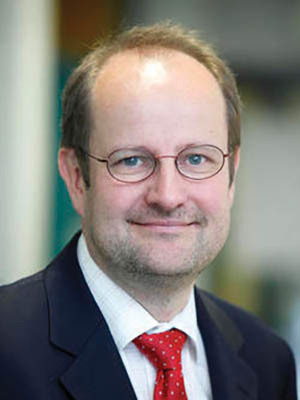
Harald Schwalbe. With stronger NMR magnets, Harald Schwalbe will be able to understand, on the structural level, why drugs work.
While Budinger advocates for more powerful MRI magnets, over in Germany, Harald Schwalbe has campaigned for record-breaking NMR machines, which get clearer signals from atoms with higher fields.
He is close to achieving that dream. The German government has funded several NMR magnets that will, when delivered, be the most powerful to date, with field strengths of 28.2 teslas. NMR magnets are more commonly referred to by their frequency — in this case, 1.2 GHz, which is the resonant frequency for hydrogen atoms at 28.2 teslas. One of the new 1.2 GHz instruments, which are being built by Bruker Corp., will land at the Goethe University Frankfurt, where Schwalbe is a professor of chemistry and biochemistry.
Over the years, Schwalbe has watched more NMR science emerge with each jump in magnetic strength, from 600 MHz to 800 MHz to, most recently, 900 MHz, which, as Schwalbe put it, broke a "size barrier" that allowed scientists to probe the largest of proteins.
"Now the question is," Schwalbe said, "what will happen when we go to 1.2 GHz?"
He has a few ideas.
Schwalbe said the 1.2 GHz will shed more light on what goes on inside a cell, such as how they build proteins or ribonucleic acid (RNA). In the latter process, cells add about 20 new nucleotides to the molecule per second. Due to limited field strength, current instruments aren’t sensitive enough to keep up with that pace.
"If we want to get real in these kind of things, I have to actually increase my sensitivity toward the speed at which cellular machines work," said Schwalbe. "That's another dream of NMR."
Yet another NMR dream is developing better pharmaceuticals. High-field NMR could not only show if a drug works, but also reveal details of its interaction with the target.
"With these machines," said Schwalbe, "we'll be able to actually understand, on a structural basis, how come the drugs work the way they work."
A new NMR magnet at the National MagLab bodes well for Schwalbe's dreams.
At 1.5 GHz (35.2 teslas), the Series Connected Hybrid (SCH) has a field strength even higher than the coming 1.2 GHz machines. But because it is a hybrid, its field is less uniform than the all-superconducting 1.2 GHz instruments.
Still, the field's uniformity is better than in physics magnets, and very effective for looking at solid biological samples, which don't demand as stable a field as solution samples. Since coming online this year, the SCH has generated impressive data that buttress the case for more strong NMR machines, said Bill Brey, a National MagLab physicist overseeing instrument development for the SCH.
Because of its very high field, the SCH can detect atoms that, until now, have been invisible to NMR, notably oxygen. The ability to observe oxygen in molecules is a game-changing capability that will allow scientists to watch the body in action at the molecular level.
"Oxygen has a number of interesting things about it," Brey said. "It's real biochemistry, not just structure — right in the active places on the molecule."
Oxygen, neurons, drug targets, weird electron behaviors: just a few of the mysteries atop scientists’ most wanted list. With the higher fields promised in next-generation magnets, researchers say, more dreamed-of discoveries will land squarely in their crosshairs.
Story by Kristen Coyne